David P. Boden
Encell Technology, Inc.
Standby battery systems have been an essential part of modern life for many years. Initially used to support the telephone system by the Bell Telephone Company they have now become necessary in virtually every walk of life. Batteries are used in series strings to provide a matching voltage with the load and they can be connected in parallel to provide the necessary capacity for the required backup time. Because they provide emergency power to critical equipment it must be assured that their batteries are maintained at full charge. This is done by continuous charging at a constant voltage, a process called float charging.
In order for the user to know the state of health of his batteries they must be monitored continuously to determine that they are healthy. The benefits of standby system monitoring are well recognized and a variety of equipment for this is now available from several suppliers. Such variables as voltage, temperature, current, AC impedance and DC resistant are measured, which can be used to assess the state-of-health of the batteries.
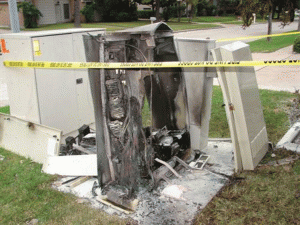
This data can be stored and transmitted to the user to provide trends that allow an estimate of remaining battery life to be made. This allows actions to be taken such as inspecting, testing or replacing batteries before failure takes place. The cost of a battery failure can be very dramatic; for example, failure of a battery costing as little as $250 can result in hundreds of thousands of dollars in consequential costs. Approximately 62 percent of cell tower failures are power related and 80 percent of those are due to battery problems. A photograph of a cell tower cabinet that was burned down by a battery fire is shown in Figure 1.
At the fundamental level some form of remote monitoring is essential. It is simply too expensive to send maintenance technicians into the field to examine and test batteries. Additionally, on-site battery capacity testing actually reduces the life of batteries and results in system down time while the battery is being discharged and recharged. The optimum level of testing and monitoring is still under debate but the need for some form of monitoring is widely recognized. However, the data that battery monitors collect are worthless unless they are analyzed regularly and trended over time to develop predictive analysis of which batteries need to be replaced and when. Because battery failures can sometimes be unpredictable the data must be reviewed daily requiring skilled personnel.
Battery monitors are widely used and have been successful in identifying deteriorated batteries but they do nothing to solve the principal causes of short battery life. A new concept is needed that controls how batteries are operated so that the inherent failure mechanisms that occur when they are operated in a state of continuous charge are eliminated. A solution is needed that automatically takes action to detect and isolate defective batteries from service before they can degrade other batteries in the string and cause the backup system to fail. This article describes a new innovative solution that manages how standby batteries can be charged to guarantee maximum life. It also tests and isolates defective batteries from service, monitors essential data and transmits it to the system owner.
Negative Effects of Float Charging
The universally used process for maintaining standby batteries in a fully charged state is by continuous constant voltage or float charging. The charging voltage is set at a value where sufficient current is flowing through the batteries to overcome parasitic self-discharge arising from the inherent thermodynamic instability of lead-acid batteries, the effects of impurities that enhance gassing, and cell-to-cell chemical or manufacturing variation. Although effective, floating also introduces some problems, especially in valve regulated lead-acid (VRLA) batteries. For example, it increases the battery temperature as a result of i2r heating and oxygen recombination[1]. It also maintains the electrode potential of the positive plates in the region where grid corrosion takes place[2]. The combined effects of high electrode potential and temperature significantly increase the rate of grid corrosion.
Temperature has a significant effect on battery life and has become a major issue because there has been a tremendous increase over the last twenty years of batteries deployed in uncontrolled outdoor installations. Temperature affects battery life because of the acceleration of electrochemical processes as temperature is increased. For every 10°C increase in temperature the life is halved. The float current, water loss and rate of corrosion of the positive grid alloy all increase. Most significant for the life of a VRLA battery on float is the combined effect of temperature on grid corrosion and water loss.
Grid Corrosion
This is a function of the charge voltage (polarization) applied to the positive plate. In float charging a constant charge voltage is applied to the battery from the charger and is distributed between the negative and positive plates. In a VRLA battery during float charging oxygen evolved from the positive plates is reduced at the negative plates. If the efficiency of this process is high the negative plate becomes depolarized. In this case most of the polarization will occur at the positive plate. Consequently, the positive electrode polarization will increase and accelerate positive grid corrosion[2].
Gas Evolution and Dry-Out
Lead-acid batteries are thermodynamically unstable which causes them to self-discharge and evolve gas whether on open circuit or charge. In a VRLA battery under an ideal situation oxygen that is evolved from the positive plates during charging is reduced to water at the negative plates. However this does not completely eliminate gassing because of two factors. First, hydrogen gas is still evolved from the negative plate at a rate equivalent to the rate of grid corrosion at the positive plate. Second, the oxygen recombination rate is not always 100 percent depending on a number of factors such as separator saturation and valve leakage. If all of the oxygen is not recombined it has to be vented. Therefore it is inescapable that if the battery is being continually charged it is continually gassing and drying out.
Equalization
An additional problem with float charging is that the electrochemical performance of the batteries in the string is not equal as a result of manufacturing variability and variability in the purity of active materials. Since the string voltage is the average of the individual cells some will have higher voltages than others. This can result in undercharging in some cells and overcharging in others. This leads to lack of equalization and drifting apart of individual capacities and early failure.
A New Concept
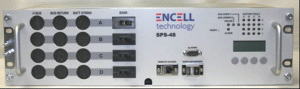
Float charging contributes to short battery life primarily as a result of its acceleration of grid corrosion and dry-out and its lack of equalization. These are all inevitable consequences of continuous overcharging. This has been well known for many years and, in fact, it is normal practice in automotive and traction applications of batteries to charge them only when necessary and then only for as long as necessary. Overcharging is considered an evil to be avoided as much as possible. We have applied this principle to the charge maintenance of standby batteries in a solution named Sentinel[3]. This is an intelligent battery management system that maintains standby batteries in a fully charged condition in a completely new way. A photograph is shown in Figure 2. The Sentinel is connected across the rectifier bus and is operated by it, therefore no separate power supply is needed.
The Sentinel operating principles are as follows.
(a) The batteries are no longer continuously overcharged. Instead they are charged periodically and only for as long as required to bring them to full charge. This assures that the minimum amount of charging is used. They are maintained in a standby mode until a designated time each day by isolating them from the rectifier bus. This prevents them from being charged while allowing them to be discharged when needed. During the standby period there is no temperature increase and gas evolution and grid corrosion are virtually eliminated. The benefit of this is that the battery life is significantly increased. The absence of heat generation considerably reduces the cooling load on the system where the batteries are installed in cabinets or buildings. Removing the batteries from float and consequent heat generation virtually eliminates thermal runaway.
(b) The Sentinel constantly reads the rectifier output voltage an immediately allows battery discharge to the load when grid power is interrupted or the output voltage sags.
(c) At the designated time each day the batteries are placed on the rectifier bus and given a maintenance charge until they are fully charged. This replaces any capacity losses due to self-discharge and minor discharges. A dv/dt type charge control is employed where the batteries are given a charge for several minutes and then allowed to stand on open circuit for several minutes. Individual battery voltages are recorded at the end of this open circuit period. This sequence is repeated until the voltage at the end of the open circuit period has increased by less than a few millivolts per battery in successive charge periods. As soon as the full charge criteria are satisfied the charge is stopped and the batteries are again isolated from the rectifier. Cumulative capacity losses from small discharges during the 24 hour period are prevented by charging whenever the cumulative discharge time exceeds five minutes. This eliminates any possibility that the batteries are allowed to stand in a discharged condition. We have compared the energy consumed by this process and by continuous overcharge and found that daily energy consumption is reduced by 86 percent.
(d) In a multistring application the strings are charged sequentially to assure string to string equalization. There is no parallel charging therefore each string is brought to full charge independent of the other strings in the system.
(e) Once all the battery strings are charged they are given a pulse discharge to test their state of health. This is done by switching a resistor across the battery terminals. The voltage/time transient is measured during the discharge and during the subsequent open circuit period, therefore the voltage change is recorded and DC resistance is calculated using the period of rest technique[4]. This eliminates such complicating factors as frequency dependence and harmonic interference often seen with AC methods. Since the voltage change after discharge termination is measured in <10 milliseconds the contribution from capacitance is negligible.
The voltage change during the test discharge is an indicator of the battery’s state of health. We have found, based on laboratory testing of batteries with different states of health, that a voltage change of >0.75 V is indicative of failure. This can be used to inform the user that the battery should be replaced.
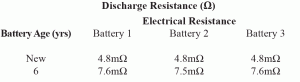
Table 1 shows DC resistance values collected from batteries with different lengths of service. The new batteries were tested immediately after installation and had resistances of 4.8 mΩ. Resistances from batteries that had been in service for some time were higher, as expected. Batteries that had been in service for six years had an average resistance of 7.53 mΩ.
(f) Following the discharge test the batteries are again placed in the standby mode.
This sequence of tests is designed to ensure that the batteries receive the minimum amount of charge consistent with returning them to full state of charge at the end of each day. It eliminates the battery-destroying mechanisms that cause premature failure, reduces their temperature since heat-generating processes are no longer occurring and eliminates plate polarization. Consequently, grid corrosion, gassing and plate degradation are significantly reduced resulting in much longer battery life. The end result is a major reduction in the cost of operating battery standby systems.
Life Testing
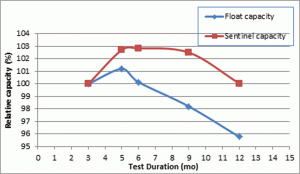
Life testing has been underway for 12 months to compare the capacity retention of batteries on continuous float and managed by the Sentinel. These tests are being carried out at 40°C (104°F) to accelerate failure. Periodically, the batteries are removed from testing and their capacity determined at the eight hour discharge rate. They are then placed back on test. The results obtained to date are shown in Figure 3. It is clear that the batteries being maintained by the Sentinel are giving higher capacities than those on float and that their rate of capacity decline is slower.
Remote Management
The Sentinel is equipped with a comprehensive data collection and remote management package. The system’s Network Management Module acts as a control and command suite for DC Plant assets. As an example, with a click of a button a Network Operations Center can initiate a system wide test of every battery instrumented in the network. The data can be delivered to the user’s laptop or mobile device via a series of easily understood dashboard displays with drill down capabilities, and can also be tightly integrated within a Carrier’s OSS system. It stores charge, test, electrochemical resistance and state of health data which are used to develop critical information including battery status, expected lifespan.
This information allows predictive maintenance planning. The data are delivered to the user’s laptop or mobile device via a series of easily understood dashboard displays. These displays are configurable to show, for, example, an entire network indicating the health of every battery, or a single battery in a particular location.
The Sentinel allows the user to access every battery in the system. For example, it can show the trended data from the voltage and state of health history of a single battery.
Conclusion
The damaging effects of maintaining VRLA batteries at full capacity by floating (continuous overcharge) can be eliminated by a more intelligent charging protocol of charging only when required and for only as long as required to restore the capacity to full charge. Replacing continuous overcharging with periodic (as required) charging eliminates heat generation so that thermal runaway is eliminated both as a failure mechanism and a safety issue. Reduction in battery temperature reduces the rates of grid corrosion, dry-out and plate degradation. This leads to longer battery life. The periodic charge algorithm also reduces energy consumption by 86 percent. We have developed a simple but effective test to determine battery health that is performed automatically and which does not require that the batteries be removed from the system for testing. It can detect under-performing batteries before they fail so that they can be replaced before they impair system capability. These benefits significantly increase the reliability and life of standby battery systems yielding considerable cost savings.
The equipment described in this article is provided with comprehensive monitoring and data display features. These allow an owner to access any battery in their system from an internet-connected computer or mobile device. This is a valuable tool to asses overall system reliability at a glance and to plan and focus maintenance activities on already diagnosed problems.
References
[1] D. Berndt, Valve Regulated Lead-Acid Batteries – Gas and Heat Management, INTELEC Conference 1988, p89-96.
[2] L. T. Lam, Advanced Lead-Acid Battery Consortium, Project N 3.1, Annual Report, 2001.
[3] Encell Technology, Inc., 12887 Hwy 441 North, Alachua, FL 32615. ( encell.com.)
[4] F. Huet, Journal of Power Sources, 70, (1998) p59-69
For more information, please visit http://encell.com.