Ron Demcko • AVX Fellow
Patrick German, Field Applications Engineer • AVX Corp.
The continual addition of smartphone features and functionality, combined with users’ growing dependence on them for both business and personal use, has made battery life and reliability increasingly vital. The digital transmission signals that these devices rely on to operate require quick pulses of current from the battery. However, these pulses can also cause the battery’s instantaneous voltage to drop below the phone’s minimum operating voltage, which can temporarily interrupt the flow of power from the battery. To solve this problem, engineers performed a series of tests on multiple battery chemistries to determine whether placing a supercapacitor in parallel with the battery could effectively improve the life of the battery and the quality of the power it provides.
The Trouble with Batteries
Battery life can be a significant source of frustration for people who rely on their smartphones to execute an ever-expanding number of personal and professional tasks, as even the latest and greatest of these devices have a relatively short battery life and require daily charging. The battery life of most smartphones under heavy use conditions (e.g., extended video recording or playback, streaming music and data, numerous active and background applications, etc.) ranges from five to 20 hours, or less than a day1.
Rechargeable batteries, like those used in smartphones and other handheld devices, begin to have trouble maintaining a charge as the batteries age, and certain battery chemistries, such as nickel/cadmium (NiCd) or nickel/metal hydride (NiMH), can develop a memory that degrades the maximum charge that’s able to be achieved over the lifetime of the battery. Additionally, most rechargeable batteries, regardless of their chemistry, are limited to a certain number of charge and discharge cycles before their maximum charge capacity begins to diminish.
Replacing a smartphone battery that has a reduced maximum capacity can be quite expensive, costing anywhere from $30 to $130, depending on the type of phone and battery2. To reduce the overall cost of smartphone ownership and improve upon their convenience, engineers have experimented with several circuit designs intended to increase the reliability of smartphone batteries. Employing a supercapacitor in parallel with the battery has proven to be one of the most effective such design developments, as this arrangement successfully improves both talk-time and overall battery life by allowing the battery to have a more constant draw than is normally experienced during GSM Pulses.
GSM Pulses: Digital Transmission
Smartphones operate using a digital transmission signal called Global Systems Mobile (GSM). GSM signals operate in two frequencies: 900 MHz and 1,800 MHz. These signal bands are comprised of hundreds of frequency bands split into 200 kHz increments, and each of these frequency bands are further split into eight segments to allow multiple conversations on a single band3.
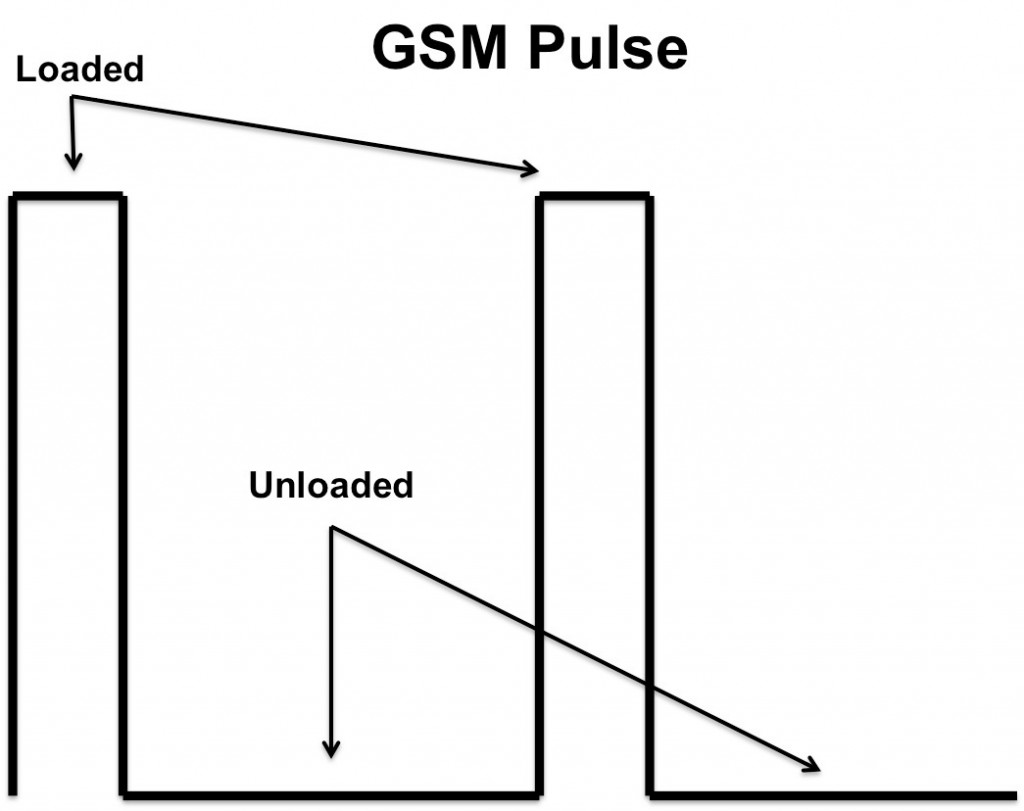
When a smartphone processes a call, the digital transmission operates within its specified frequency with a short-burst duty cycle of 0.125, which strains the battery a lot more than analog signals normally do3. In digital systems, like GSM, the transmissions occur in short pulses. Consequently, the batteries in such systems only produce power for the purpose of transmission for one-eighth the duration of any given GSM signal, which can cause the battery to experience quick pulses of energy and respond with sudden spikes of output current instead of the slow, controlled output that’s common to analog transmission.
The amount of current required of a smartphone battery during these short pulses determines whether or not the battery can continue to provide enough power to operate the phone. If the amount of current required of the battery during GSM pulses is too high, the battery will experience a brief reduction in voltage to provide the necessary current. Although effective, this compensation could potentially cause the battery to drop below the phone’s minimum rated operating voltage, interrupting power to the phone.
Test Conditions
The following experiment was developed to simulate the effect of these quick pulses on the overall talk-time of a smartphone battery. The tests were run in two circuit topologies, one with a parallel supercapacitor and one without (Figure 2), and data was collected and recorded during both the loaded and unloaded portions of the GSM signal test (Figure 1).
In order to record the effects of GSM pulses on rechargeable smartphone batteries, engineers developed a test circuit that would allow for the alteration of frequency, duty cycle, and load conditions without major alterations to the physical circuit, and simulated a GSM pulse.
The schematics in Figure 2 illustrate the circuit topology used in the tests. The GSM pulse was created using a software-controlled switch, realized using a MOSFET and digital controller, that could simulate the pulsed loading of a smartphone battery. The switch routed power through a variable load, realized in the schematic as a potentiometer, and the variable resistor provided the ability to test the circuit at a variety of loading conditions.
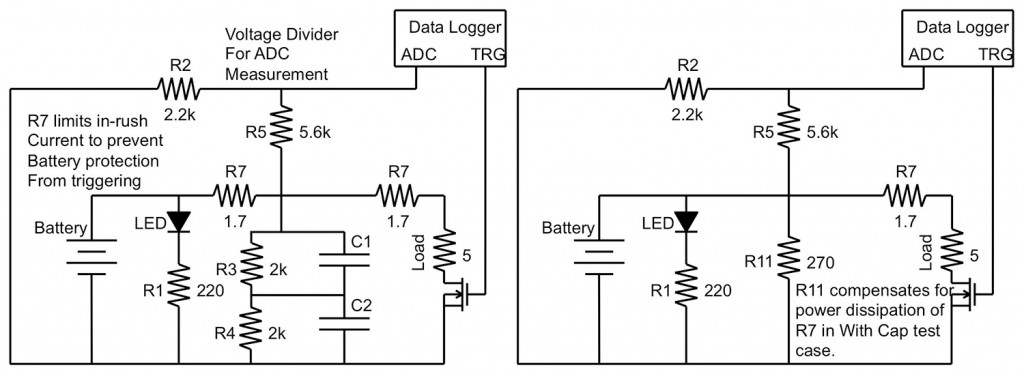
This setup allowed a high-speed software controller to alter all aspects of the circuit while taking test measurements from both the loaded (high signal) and unloaded (low signal) portions of the simulated GSM pulse, as well as significantly reduced the possibility of human error affecting the test outcomes.
Battery Chemistry and Preconditioning
Three different battery chemistries were studied in this test: NiMH, NiCd and Li-ion. Each battery had different power and energy ratings, so engineers obtained two of each battery type so they could more effectively test the effects of parallel capacitance, rather than the strength of one type of battery chemistry.
The AA NiCd batteries were connected in a three-cell stack that resulted in a power supply of 4.5V, 1,000 mAh. The AA NiMH batteries, also connected in a three-cell stack, had a power supply of 4.5V, 2,800 mAh, and the AA Li-ion batteries, connected in a two-cell stack, had a power supply of 4.5V, 750 mAh.
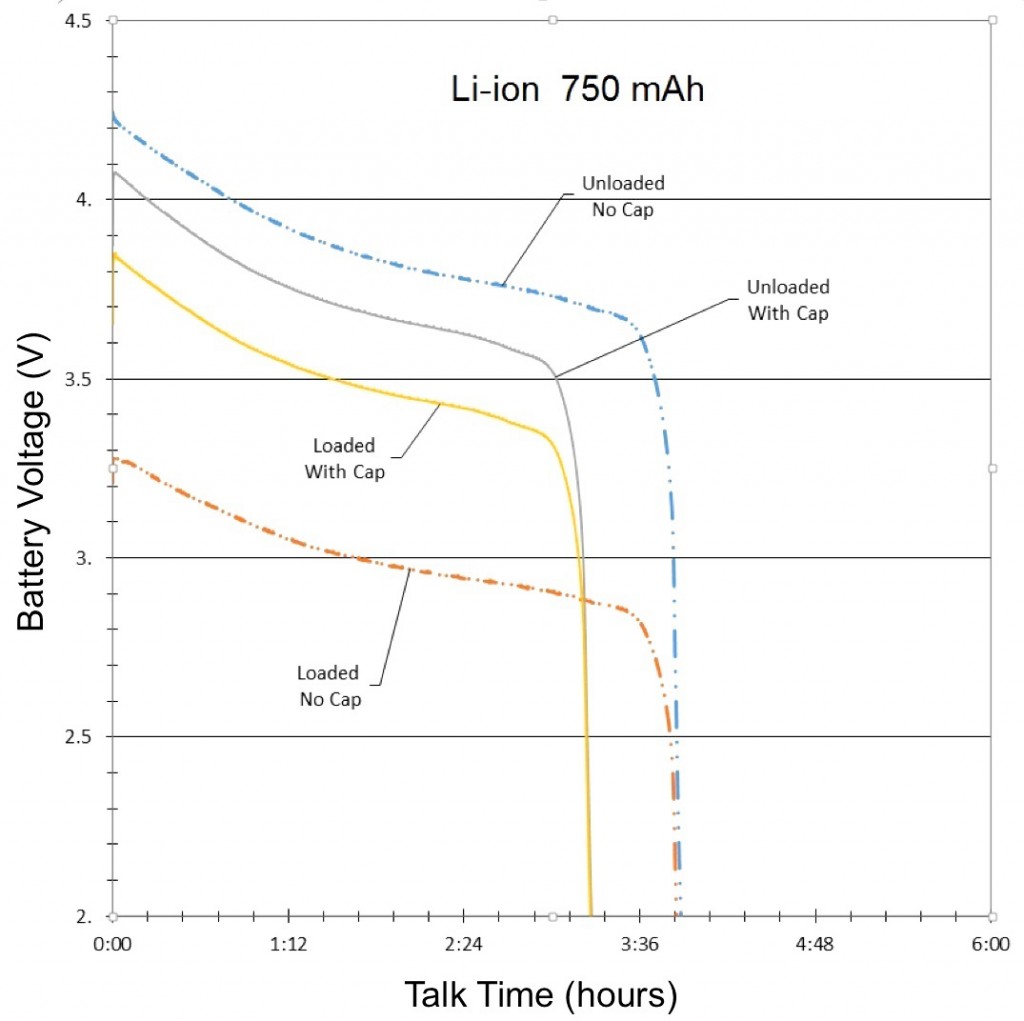
Before testing, each battery stack was run through three full charge and discharge cycles. The batteries were charged to full capacity using commercially-available battery chargers, and were then discharged by a means that simulated a loaded smartphone battery left in an infinite loop until the battery was fully discharged.
Testing
Once the charge and discharge cycles were complete, the batteries were fully recharged and discharged through identical loads maintained by the software controller. Each battery was tested in two conditions: one in which the battery was placed in parallel with a 5F supercapacitor, and one in which the battery did not have a parallel supercapacitor.
The results from these tests were recorded individually, and then graphed based on battery chemistry.
Test Results
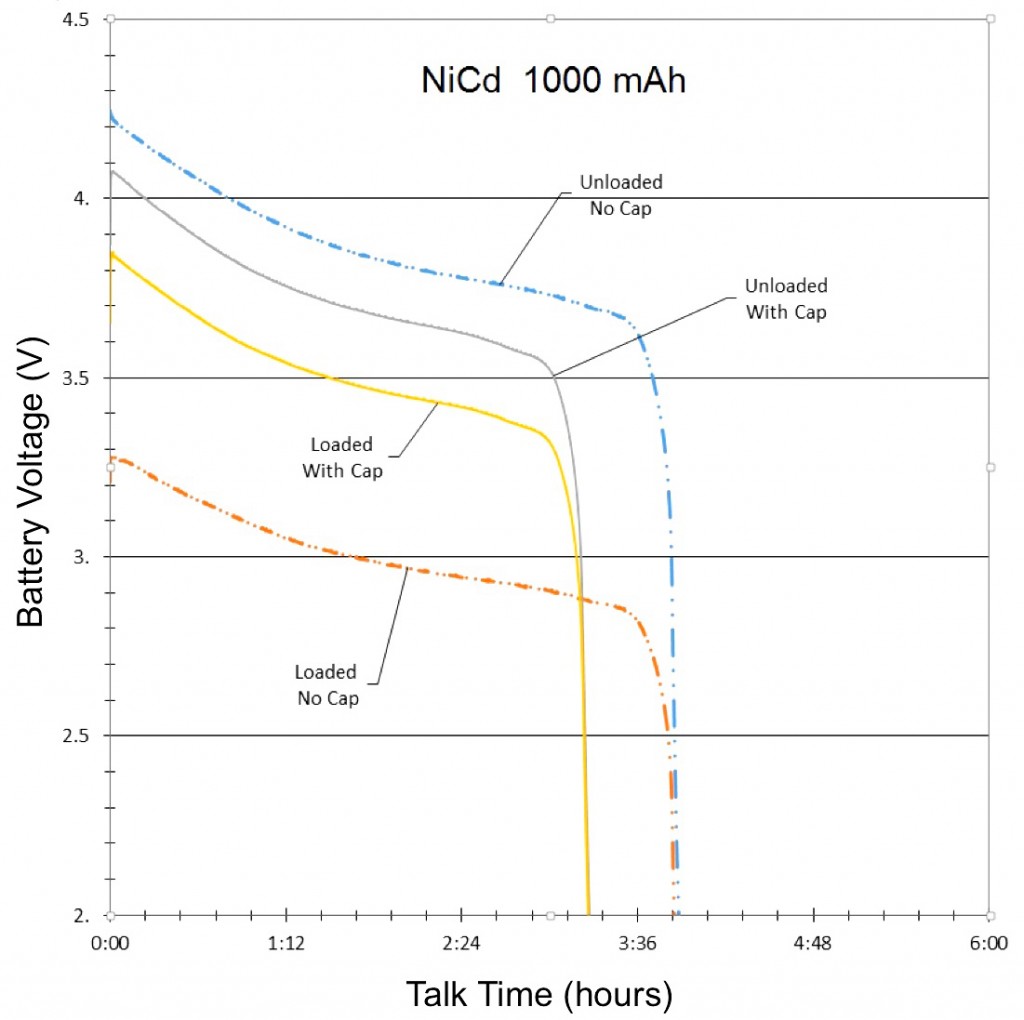
The final results of the NiMH, NiCd and Li-ion tests were compiled and graphed (See Figures 3, 4, and 5). Each graph is separated into four lines, based on test and signal conditions. These conditions are: Unloaded Without – Battery not shunted to the load, and battery has no parallel capacitor; Loaded Without – Battery is shunted to the load, and battery has no parallel capacitor; Unloaded With – Battery is not shunted to the load, and battery has a parallel capacitor; and Loaded With – Battery is shunted to the load, and battery has a parallel capacitor.
The most apparent benefit of the addition of the supercapacitor was the drastic change in voltage difference between loaded and unloaded conditions. When the batteries were not paired with a parallel supercapacitor, their voltage dropped tremendously when forced to provide the sudden spikes of current demanded by the GSM transmissions. Alternately, when the batteries were paired with a parallel supercapacitor, their voltage remained relatively stable, as they were not required to supply the entirety of the current for the GSM pulses. The low ESR and ESL of the supercapacitor allowed it to react to the sudden current demands of the GSM pulses more quickly than any of the batteries alone.
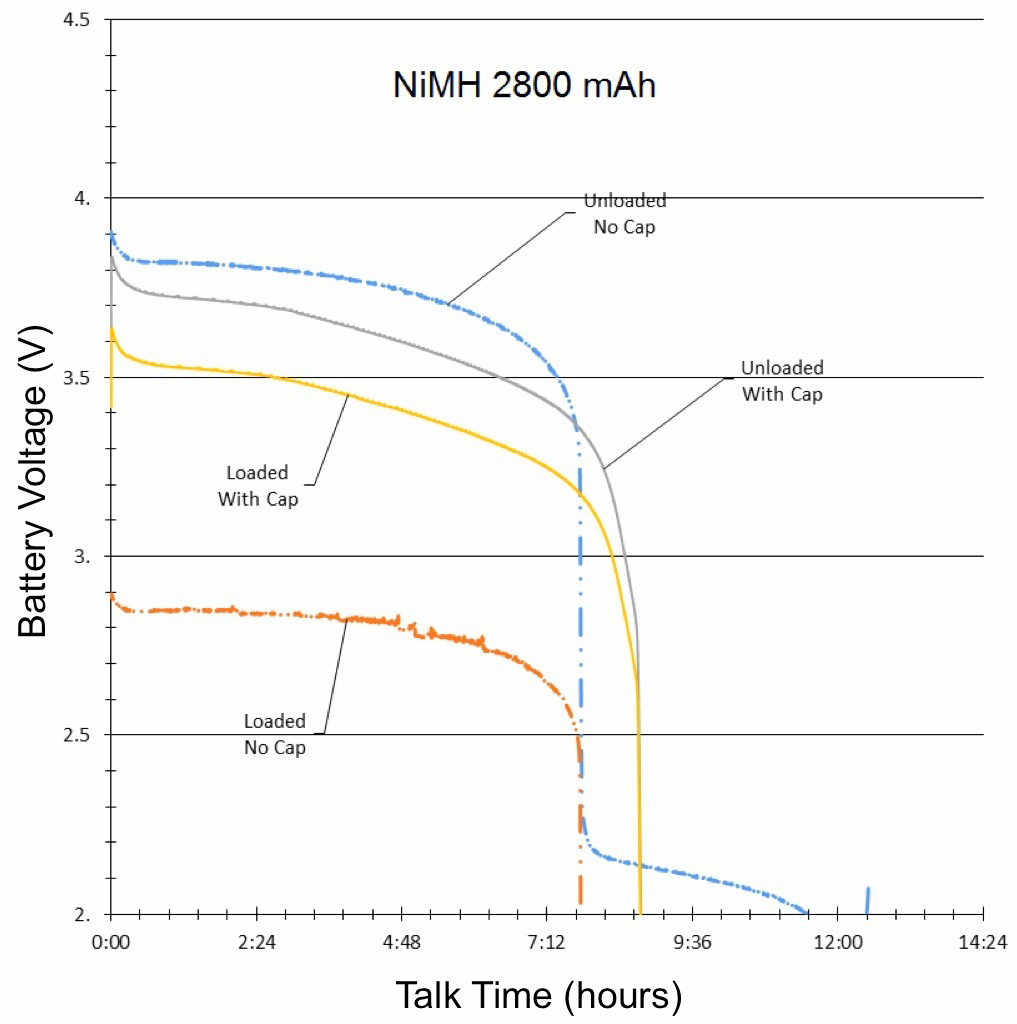
Additionally, since the parallel supercapacitors prevented the voltage of the smartphone batteries from dropping so significantly in response to GSM pulses, they also prevented the batteries from dropping below the average smartphone’s minimum operating voltage, which effectively extended the talk-time of the phone.
Final Notes: Sizing the Capacitor
The amount of capacitance required to achieve the extended battery life and talk-time benefits enabled by a parallel supercapacitor depends on the specific application at hand. If a particular smartphone relies on the battery to provide constant background power and only relies on the supercapacitor for pulse support, the supercapacitor should be sized to supply the energy required by the pulse to prevent the battery from experiencing the voltage drop it would have experienced as the only power source.
Alternately, for smartphone applications that use the supercapacitor to merely reduce the voltage drop of the battery, the capacitance of the supercapacitor isn’t as important as its ESR. In this case, the ESR of the supercapacitor in parallel with the internal resistance of the battery will ultimately lead to a lower Thevenin resistance than what would be seen in either unit alone, effectively reducing the voltage drop when power is pulled from the system.
References
1. Byrne, K. (2014, November 21). Best battery life 2014 – 60 smartphones tested. Retrieved May 28, 2015, from
http://www.expertreviews.com.uk/mobile-phones/1402071/best-battery-life-2014-60-smartphones-tested
2. Battery | Geek.com. (n.d.). Retrieved May 28, 2015, from http://www.geek.com/smartphone-buyers-guide/battery/
3. Steer, W. (2001, February 1). GSM phone signal analysis. Retrieved May 28, 2015, from http://www.techmind.org/gsm
For more information, contact AVX Corp. at www.avx.com.