Christopher Lohmeier, R&D Engineer, Vishay Intertechnology
Tom Veik, Sr. Engineering Technician, Vishay Intertechnology
Modern automobiles need to become more efficient to comply with future fuel economy standards. Much of this efficiency can be, and has been, gained with innovations that depend on the vehicle’s electrical system. Technological innovations such as stop-start, drive-by-wire, and brake-by-wire systems are just a few of these improvements. All of these new technologies, however, have one major downside in that they rely on the one component of the electrical system that has not seen much innovation since the 1950s, the lead-acid battery. Proper battery management will be the key to allowing innovation to continue in future designs, and it can be achieved with an intelligent battery sensor (IBS). An IBS unit gives precise and on-demand current, voltage and temperature (IVT) measurements from the battery. This information allows for accurate “state of charge” and “state of health” calculations to be performed, ensuring the electrical system works at the highest level of efficiency.
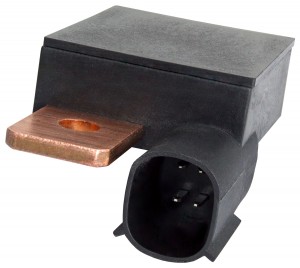
IBS Overview
An IBS is a total measurement system for lead-acid battery management. These components measure the charge or discharge current flowing through the battery, the voltage across the battery terminals, as well as the temperature of the battery through thermal conduction between the battery post and the IBS unit itself. All three of these measurements are taken almost simultaneously to ensure accurate measurements, even during times of rapidly changing conditions. An IBS can use a LIN communication protocol to send the results of these measurements to the vehicle’s electronic control unit (ECU) or other control system. LIN is a robust protocol with a great noise tolerance for automotive environments. A LIN bus is already available on most new production models, or can easily be developed with simple microcontrollers when using the IBS in other automotive or non-automotive applications.
IBS units should be built to handle the full range of automotive operating conditions. For example, a –40°C to 115°C temperature rating would allow the device to survive conditions that would damage even the newest, most advanced lead-acid batteries. In addition, a high voltage range lets the unit continue to retrieve data under both battery overcharge and undercharge conditions. The device should be able to monitor the full current band at both ends of the voltage and temperature extremes with minimal loss in accuracy.
IBS Accuracy
At the heart of an IBS is a shunt, which is specifically designed for sensing battery currents. The precision internal electronics of the IBS should be robust enough to handle the under-hood environment of modern vehicles and maintain accuracy when sensing the voltage drop across the shunt. The IBS and integrated electronics must handle all 12 V automotive starting currents, while limiting the error to a maximum of 0.5 percent (±30 mA offset) over the entire measurement and battery temperature range.
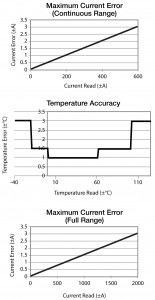
An additional benefit of an IBS, on top of the current sensing ability, is that the battery temperature sensor and voltage sensor are all contained within the one unit. So, for example, let’s say that the voltage measurement of an IBS offers accuracy of ±50 mV over a range of 4 V to 18 V through the entire battery temperature range, while the battery temperature sensor has a maximum error of 3°C at the outer edges of the total range. The upper temperature range for a lead-acid battery is generally considered to be 60°C and a practical lower limit is no less than 0°C for a running vehicle. Within these practical ranges, our example IBS has a temperature error of no more than 1°C (more information in Figure 2). This is accurate enough to set shut-down warnings and to define current limits for the battery under all operating conditions. Having all of these sensors contained in one single device eliminates the need for extra sensors or other cost-adding systems to retrieve this information. All three measured values are returned in one data packet over the LIN bus, ensuring the IBS provides accurate, real-time, and correlated measurements of all battery parameters.
Automotive Applications
Modern Day Automotive Electrical Loads
Knowledge of the battery’s health and charge are very important in modern day vehicles. Innovations such as stop-start, drive-by-wire, and the conversion of once hydraulic systems to electrical systems add an even greater load to the vehicle’s electrical battery system, which motorists are already relying on for the safety of themselves and others around them. An IBS allows the vehicle to prioritize all of these electrical loads on a scale from “comfort related” to “safety critical.” The automobile can then shut these systems down in a logical order to warn drivers of an impending battery problem, while keeping them safe.
Stop-Start Technology
Stop-start technology has been implemented on hybrid vehicles for many years and is now starting to be implemented as a standalone system on conventional internal combustion engine (ICE) powered cars. One significant problem that still needs to be addressed with stop-start technology, however, is the 12 V battery system. Just the additional number of restarts on a normal trip through town would be enough to drain and possibly destroy a conventional lead-acid battery, which is why most stop-start enabled vehicles use absorbed glass mat (AGM) batteries. While AGM batteries enhance a car’s ability to use stop-start technology, problems still persist with systems that need to continue to run after the car shuts itself down, including the ECU, safety monitoring, lighting, navigation, climate control and general comfort systems. All of these systems present a huge drain on the car’s battery that can destroy it if not monitored closely. Current stop-start models address this by either turning off the comfort systems while the car is stopped, or by routinely restarting the engine to ensure the battery is kept charged throughout the stop. A proper battery management sensor could better ensure that the battery was operated at safe levels only. This would increase fuel economy even further by forcing the car to restart when, and only when, it was absolutely necessary during a stop. It could even allow the vehicle’s comfort systems to be intermittently pulsed on and off as needed without an engine restart when the battery condition was in a known safe operating area, giving the driver a more comfortable in-car experience.
Hybrid Vehicles
There are two main categories of hybrid vehicles: series hybrids and parallel hybrids [4]. In a series hybrid the ICE does not actually propel the vehicle forward; instead it is used to power an electric generator and charge any on-board batteries. In a parallel hybrid both the electric motor and the ICE are connected to the drive train. This allows both devices to power the motor at once when there is high demand, or just the electric motor when there is low demand. This mode allows the ICE to use the electric motor as a generator and recharge the hybrids batteries when needed. Both designs can have an included high-voltage battery pack to store power for the electric drive motor. To get the most efficiency out of each design this battery back needs to be closely monitored by an accurate battery management system.
Electric Vehicles
Electric vehicles are based off a fully electric drive system with no ICE contained on the vehicle at all. In a fully electric vehicles (FEV), batteries on the car or truck itself provide the only power received by the electric drive motor and all the standard electrical systems. A good battery management system is more important in this type of vehicle than in any other. If the energy in the batteries is depleted, there is no back-up. The batteries in FEVs are usually arranged with cells in series or parallel stacks to acquire the needed output voltage. Each one of these stacks should contain its own battery management system to ensure that one individual cell failure will not bring down the whole battery system.
Other Sensing Technologies
Open-Loop Hall Effect Sensor
Reliability is one of the issues that makes many other monitoring technologies undesirable in automotive applications. Due to cost, size, and measurement range, the open-loop Hall Effect sensor is really the only comparable battery monitoring technology. This sensor uses the Hall Effect, where a magnetic field is formed around a current-carrying wire to measure the current flowing through that wire. A current transformer would not work with the DC automotive electrical system, and a closed-loop Hall Effect sensor would be far too costly and large at the current values being measured [1]. The greatest attribute of the open-loop Hall Effect sensor is that since it is not actually in the current path, there is no power loss while current is being measured; however, this comes at the expense of accuracy and reliability [1].
Issues Associated With Shunt Sensing
Given that an IBS does use a resistive shunt at its core for current measurements, there is a loss associated with it being in-circuit. However, by incorporating shunts with extremely low resistance values, this loss can be negligible over most of the current range. For example, a 100 µΩ shunt only causes a loss of 1 W at 100 A of current. That is a 0.083 percent power loss if you have 100 A being sourced by a 12 V battery. At the current values observed in the real- world test, the shunt loss is 900 µW with 3 A of standard running current, and 12.25 W at the short-lived maximum starting current spike of 350 A. On the real-world test, the 35-inch, 4- AWG positive battery cable has a resistance of 788 µΩ [2]. This means there is nearly eight times more power loss in just the positive battery cable then there is in the IBS. The use of such a low-value shunt should permit the IBS unit to operate at a current range of ± 600 A continuously and ± 2,000 A in pulsed applications not exceeding 900 J.
Since the Hall sensor is not directly connected to the current-carrying wire, outside forces can cause significant error in the magnetic field measurements [1]. The earth’s magnetic field alone can cause a 0.4 A error, not to mention the fields generated by other coils, conductors, and electric motors / generators internal to the vehicle [1]. Being in-circuit means an IBS allows far fewer errors from outside interference compared to a Hall Effect sensor. The maximum current sensing error under any in-car condition for an IBS unit should be 0.5 % plus offset (30 mA), which is the same error that can be observed due to the earth’s magnetic field in the Hall Effect sensor just by changing directions with 80 A of current flowing [1].
Open-loop Hall Effect devices have a natural offset associated with them that is present even at zero current [1]. This offset is greatly influenced by temperature; even good sensors have a standard shift of up to 0.5 percent. An additional temperature sensor is needed to attempt to account for the offset change [1]. A final disadvantage of the Hall Effect sensor is that it may require an in-circuit calibration due to the output relying so heavily on the position of the sensor. An IBS’s current measurements are all centered on 0 A with no natural offset to account for, except noise. Resistive shunts can have a temperature coefficient of resistance (TCR) that would cause errors in readings over the wide temperature span that an IBS is rated for. Through processing techniques and use of the temperature sensor already onboard, this coefficient could be calculated out and would only have a minimal effect on measurements from the device, never exceeding the rated accuracy. These and other calculations all come preloaded on the IBS unit, so it is truly a plug-and-play device with no secondary or in-system calibration needed.
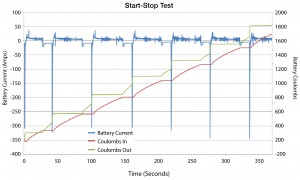
Real-World Observations
A real-world city driving test was performed using an IBS, which was attached to the negative battery post as it would be in any automotive application to monitor the battery. Two separate driving tests were carried out in the same manner. The route taken was around city blocks in Columbus, Nebraska. This route was chosen in order to obtain an approximation of a standard morning commute, without interrupting the flow of traffic and without having the test broken up by other drivers. The first of the two tests was a simulated stop-start test. This was simulated with the vehicle coming to a complete stop at pre-determined places (six stops in the 12 city block test) and shutting the vehicle off as soon as all forward motion had ceased. The stop was timed and after the 15-second stop interval the engine was turned on and forward motion was resumed. The second test was made to mimic the first one as closely as possible with one exception: the vehicle was never turned off. The stop duration was kept to the same 15-second period. The route, maximum speed, and acceleration were all monitored to mirror the first test as strictly as possible. Comparing the results of these tests shows how much more taxing a stop-start system is on a battery when compared to the standard automotive system in most cars and trucks today. The stop-start test results also show the effectiveness of an IBS as an automotive sensing system within a true automotive environment.
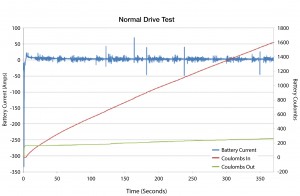
The results of both real-world driving tests are shown in Figures 3 and 4. This simple test demonstrates the need for a robust and precise battery monitoring system. Each test lasted just over six minutes and had six 15-second stops. The restarts in the stop-start test associated with these six stops took 1,528 more coulombs of charge out of the battery than the normal driving test. The stop-start test even ended with a net reduction in battery charge (135 coulombs) compared to the beginning of the test. There is an initial start on the normal driving test in Figure 4, but after the subsequent charge loss there is a net charge applied to the battery, which accounts for inefficiencies within the battery.
The test was a short approximation of a morning drive to work in Columbus, Nebraska, where traffic jams are not much of an issue and the test battery was brand new. If this was a car leaving Los Angeles or Munich at rush hour, the number of stops compared to time driving could be much worse. If a vehicle containing a weaker battery was in the stop-start case above for a longer period of time, it is easy to imagine a case where the battery’s charge may be lowered to the point where it can’t restart the car after one of these stops. If the car or truck had been equipped with an IBS, the engine control system would be able to accurately monitor the battery and determine its ability to restart the automobile.
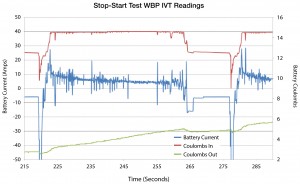
An IBS is a one-unit solution to the problem of dying batteries. The unit has the ability to accurately measure all the needed battery parameters for precise predictions of battery health. These measurements are shown in Figure 5, which is taken from the tail end of the stop-start test observed in Figure 3. The graph shows the raw data sent from the IBS that would be received by a central controller and used for battery health information.
Other Applications
IBS units are perfect for any automotive application, but also excel in many others. Most uninterrupted power supplies (UPS) contain a lead-acid battery for back-up power, and these batteries need to be monitored just as the battery in a car. Knowing the state of health in a back-up battery not only ensures the battery will work when needed, but also allows for a longer overall lifespan of the battery for significant cost savings. Golf carts, electric forklifts, and personal mobility vehicles all contain electric motors powered by lead-acid batteries. Knowing the state of charge in these batteries allows a control system to warn the user when recharge is needed. An IBS unit would also allow the system to limit current draw (i.e. by limiting the top speed on a golf cart) to further extend the remaining battery life and allow the user to travel a greater distance back to a recharge area.
IBS units are also needed in safety applications such as emergency lighting and medical beds. Emergency lighting is a battery-powered back-up light source. Monitoring the battery would allow installers to know precisely when it can no longer power the lights for the necessary time span, providing cost savings compared to the replacement of batteries on a scheduled system. The IBS would also make certain that a weak battery would be noticed and could be replaced earlier than scheduled, ensuring the lights perform as expected during an emergency. Medical beds each have a lead-acid battery back-up system that guarantees vital patient systems continue working even in the event of a power and / or back-up generator failure. If one of these batteries is in a low state of health when an emergency situation occurs, it could cost a patient his or her life. An IBS is better able to monitor the battery’s state of health than conventional sensing systems.
Renewable energy applications are another area in which IBS units excel. The first and most obvious area is anywhere a battery is charged by a renewable energy source and used as either a back-up source or the full source, such as off-grid applications and recreational vehicles. The IBS works in about the same function here as it would in a vehicle or a UPS. However, there are many more applications in the renewable energy field. One of these is in maximum power point tracking (MPPT) circuits. Solar panels output maximum power at different currents and voltages depending on conditions affecting the panel [3], and the IBS can be used to monitor the current and voltage output of the panel. The overall power output of a panel or array can be increased by as much as 30 percent over conventional systems by simply combining the IBS measurements with a MPPT algorithm and a simple converter circuit [3]. This additional output outweighs any losses observed due to the resistive sensing element [3]. The increase also greatly reduces the cost-to-power ratio for solar systems, as the panel is by far the most expensive component [3].
Conclusion
The ability of IBS units to handle harsh automotive environments makes it more than capable of being used in many other indoor / outdoor applications. This robustness and the capacity to accurately measure all parameters suit the devices to almost any battery monitoring application. Future improvements in vehicle efficiency will require greater energy management schemes in all automobiles. When included in a vehicle’s electrical system, an IBS allows further and greater technological innovations to run off the tried-and-true lead-acid battery, as well as the newer hybrid and electric vehicle battery technologies.
Reference
[1] Pettigrew, Warren. “Current Sensor Selection for Demanding Applications.” Power Electronics Europe, Issue 2. 2008: 26-28. 25 Feb. 2014.
[2] “Solid and Stranded Conductor AWG Chart.” Calmont Wire and Cable. 26 Feb 2014. Web. 26 Feb 2014. <http://www.calmont.com/pdf/calmont-eng-wire-gauge.pdf>.
[3] Lohmeier, Christopher. Highly Efficient Maximum Power Point Tracking Using a Quasi-Double-Boost DC/DC Converter for Photovoltaic Systems. MS thesis. University of Nebraska-Lincoln, 2011. Lincoln, NE: Dec 2011